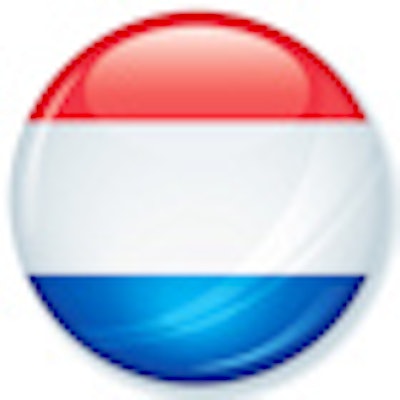
Lipid-coated gas microbubbles injected into the blood can be used as an ultrasound contrast agent for diagnosis of cancers or cardiovascular disease. However, most existing contrast-enhanced ultrasound techniques suffer from artifacts due to nonlinear wave propagation through the contrast agent.
Artifacts can impair the diagnostic quality of the resulting image. To overcome these limitations, researchers from Erasmus Medical Center in Rotterdam, the Netherlands, have developed a means of creating artifact-free images of the microbubbles, which they describe in an article published in the 7 November issue of Physics in Medicine and Biology.
The technique is based on counterpropagating wave interaction. For typical acoustic pressures employed in diagnostic ultrasound imaging, two ultrasound pulses propagating in opposite directions through biological tissue will pass over each without any noticeable effect. However, lipid-coated microbubbles within a liquid allow interaction of counterpropagating acoustic waves (Phys Med Biol., 7 November 2012, Vol. 57:21, pp. L9-L18).
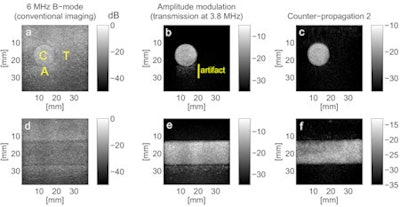
"Like microcracks in a solid material, lipid-coated gas microbubbles exhibit complex nonlinear elastic properties that allow counterpropagating waves to interact," explained biomedical engineering researcher Guillaume Renaud, PhD. "Therefore, making an image of the interaction between the two waves allows one to detect the contrast agent microbubbles and distinguish them from tissue."
In vitro implementation
To test their hypothesis, Renaud and colleagues implemented counterpropagation-based ultrasound imaging using a simple pulse sequence: transmission of a low-frequency (LF) wave immediately followed by a high-frequency (HF) wave. After the LF wave is scattered or reflected, the resulting back-propagating wave crosses the forward-propagating HF wave.
"If a subharmonic bubble vibration appears in response to the HF wave, then the subharmonic amplitude can be actively manipulated (enhanced) by simultaneously exciting the bubble with an additional LF signal," Renaud explained.
The team used an ultrasound scanner (Model iU22, Philips Healthcare) with a 3-9 megahertz (MHz) ultrasound probe to image a tissue-mimicking phantom containing a cylindrical cavity filled with a contrast agent (SonoVue, Bracco). Counterpropagation imaging was performed by transmitting a 1.5 µs pulses at 3.8 MHz and 8 MHz, firstly with equal peak negative pressures (CP1), and then with the amplitude of the HF wave roughly double that of the LF wave (CP2).
Counterpropagation images showing the location of the microbubbles were obtained by subtracting a reference signal (transmitting the LF wave without the HF wave) from the echoes received when both waves were transmitted. The researchers used a low-pass filter with a cutoff frequency of 6.2 MHz to suppress echoes arising from linear backscattering of the HF wave.
The CP1 and CP2 pulse sequences were also compared to amplitude modulation (AM), the default technique for contrast agent detection on this system. AM images were formed by transmitting a 1.3 µs 3.8 MHz wave at full and half amplitude, then subtracting the summed single-amplitude backscattered signals from the double-amplitude backscattered signals.
Transverse and longitudinal phantom images obtained using AM clearly showed a pseudo-enhancement behind the vessel due to nonlinear propagation artifacts. In contrast, no artifacts were observed for either of the counter-propagation pulse sequences.
To quantitatively compare performance, the researchers calculated maximum signal amplitudes in regions of contrast and tissue, and the region in which the artifact appeared. They then computed contrast-to-tissue and artifact-to-contrast ratios for the three techniques, as well as for conventional B-mode ultrasound images.
AM provided a better contrast-to-tissue ratio (21 dB) than counter-propagation (13.5 and 17.5 dB for CP1 and CP2, respectively). However, AM was dramatically affected by the nonlinear propagation artifact, with an artifact-to-contrast ratio of -11 dB. The counter-propagation images, on the other hand, were free from artifacts and had improved artifact-to-contrast ratios of -13 and -17 dB for CP1 and CP2, respectively.
The authors note the echogenicity of the tissue-mimicking material is weaker than that of tissue, thus the artifact appeared weaker in vitro than was seen in previous in vivo images. They expect, therefore, that in vivo counterpropagation imaging will further improve the artifact-to-contrast ratio compared with AM.
"The new technique would be beneficial to all applications of contrast-enhanced ultrasound imaging: quantification of neovascularization in atherosclerotic plaques and, more generally, assessment of tissue blood perfusion for diagnosis of tumor malignity or evaluation of myocardial tissue damage subsequent to a myocardial infarction," Renaud told medicalphysicsweb.
The team now plans to perform in vivo validation of the technique, with a focus on characterization of neovascularization within atherosclerotic plaques of the carotid artery.
© IOP Publishing Limited. Republished with permission from medicalphysicsweb, a community website covering fundamental research and emerging technologies in medical imaging and radiation therapy.