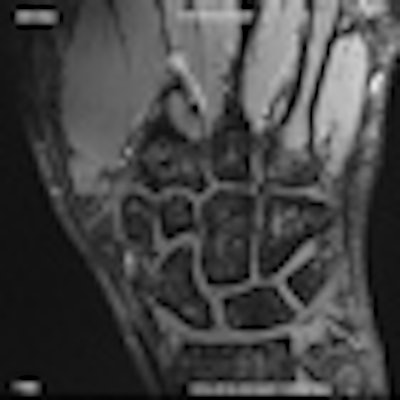
VIENNA - The standard field strength of clinical MR units is still 1.5 tesla, but the past five years have seen a tremendous increase in the number of devices operating at 3.0 tesla. Those high-field systems operating at 3.0 tesla are especially helpful in the examination of the head, spine, and musculoskeletal system. When it comes to imaging of the body, the results of trunk examinations at 3.0 tesla or above are often inferior to those gained from operating at 1.5 tesla, due to the fact that shorter wave lengths lead to artificial and inhomogeneous signal intensity and possible contrast weighting in the thorax, abdomen, and pelvis.
Besides the clinical field, whole-body systems up to 9.4 tesla have become available in experimental settings and have already proven to be significantly beneficial in special purpose examinations. Professor Fritz Schick, head of the department of experimental radiology in Tübingen, Germany, gives some examples: "One interesting field is highly resolved anatomical MRI of the brain or joints. Some contrast mechanisms are clearly more effective at higher field strength. Susceptibility-based imaging (SWI, BOLD, or T2*-imaging) significantly benefits from the ultrahigh field (7.0 tesla). Recent research has shown that functional MRI at 7.0 tesla provided better results compared to 3.0 tesla in the presurgical evaluation of patients with tumors located close to the senso-motor area in the brain. In patients with multiple sclerosis, susceptibility-weighted imaging (SWI) at 7.0 tesla reveals vein density and focal iron accumulations in MS plaques, which gives insight into the pathophysiology of this disease in vivo for the first time."
![]() |
A susceptibility-weighted image (SWI) with high spatial resolution illustrating the very small normal veins in the white matter of the brain. Note that on the left a malignant brain tumor is visible as a hyperintense lesion in the background. |
High-field MR systems at 7.0 tesla with their higher signal-to-noise ratio (SNR) also make nuclei other than protein, such as sodium (23Na), phosphorus (31P), carbon (13C), and others, visible. This is especially the case for sodium imaging, due to its rather low sensitivity, which is limited at 3.0 tesla and below, and it has proven to be very valuable in the aftercare of cartilage repair surgery. Sodium imaging correlates with the proteoglycan content of cartilage, which is a significant measure of tissue quality due to its important role in the biomechanical functions of the cartilage. Higher SNR and spectral resolution at 7.0 tesla are also responsible for a significant improvement in phosphorus spectroscopy, which allows for quantification of the energy metabolism of muscle in significantly reduced examination times in comparison with 3.0 tesla, making it a highly attractive application to be introduced to clinical use.
But just as every coin has two sides, there are also drawbacks and technical difficulties to overcome when operating MR systems at high field strengths. Some are closely connected to the very high SNR, which is normally a welcome effect that can either allow an increase in the spatial resolution within the same examination time or a reduction in the examination time while maintaining spatial resolution. However, it also comes with some unwelcome side effects. Spatially inhomogeneous RF excitation and signal yield are to be expected when RF wavelength (which is 52 cm at 1.5 tesla, 26 cm at 3.0 tesla, and 11 cm at 7.0 tesla in water) is about the size of the body part under investigation.
Specific absorption rate (SAR) on the other hand, which measures the amount of energy absorbed by the human body or a specific amount of tissue, increases with the square of the field strength.
![]() |
A collection of images in a patient eight years after autologous osteochondral transplantation. Besides a standard morphological image (A) which shows minor defects in the transplantation area, several compositional (biochemical) MR methods are presented. T2 mapping (B) is collagen and water-specific, while chemical exchange saturation transfer (CEST) (C) and sodium imaging (D) are proteoglycan-specific MR methods. All biochemical methods reveal alterations in the normal structure of the cartilage transplant. So far CEST and Sodium (23Na) imaging can only be performed with 7-tesla MR scanners. |
"This means that the same sequence would involve a four-times-higher SAR at 3.0 tesla and 22 times higher at 7.0 tesla when compared to 1.5 tesla. On the other hand, legal restrictions of SAR are not field-dependent. For this reason, there are obstacles to the use of common RF pulses, sequence types, and imaging parameters at higher field strengths. Therefore, with certain MR techniques, novel sequence programming is necessary to reduce SAR exposure in the human body at 7.0 tesla, which then allows the coverage of larger volumes during examination with consecutive reduction in scan times," said Professor Siegfried Trattnig, head of the Excellence Centre of High-Field MRI in Vienna, explaining the difficulties of clinical integration of high-field imaging.
A major effort has to be made to adapt RF pulses and sequences to the higher field strengths before imaging beyond 3.0 tesla can be made available in clinical routine. Furthermore multitransmitter RF systems will be necessary in order to overcome the problems of RF inhomogeneity in extended body parts beyond 3.0 tesla. Eventually individual adjustment of intensity and a phase of several transmitter coils could help cope with the problem.
![]() |
High-resolution anatomical-morphological image of the wrist of a volunteer, during normal examination time at 7 tesla. (All images provided by Prof. Siegfried Trattnig) |
While an increased signal-to-noise ratio is welcome, the effect of the acoustic noise originating from strong mechanical forces on the gradient coils is an issue that has to be dealt with by constructing additional noise protection systems, which will also increase the weight of MR devices.
Apart from technical difficulties and adaptations that still need to be carried out before high-field imaging beyond 3.0 tesla becomes available for clinical use, both professors pointed out that there are already fields where the irrefutable benefits can be seen, but that it will still take time before patients can experience them first hand: "Imaging of smaller human body parts (leg, wrist, ankle, knee, or brain) is already possible on high-field MR systems with extraordinarily high image quality. I would expect clinical application of 7-tesla systems for special purposes (especially brain and joints) in the near future. But I am not sure whether high-field systems will replace standard imaging at 1.5 to 3.0 tesla within the next 10 years."
The session will feature talks by Dr. Susanne Francis, who will give further insights into the readiness of 7 tesla imaging for clinical use; Dr. Michael Bock, who will go into detail on the challenges of high-field MR systems; and Prof. Mark Ladd, who will explain how to cope with the control radiofrequency fields and SAR limits.
Originally published in ECR Today March 3, 2012.
Copyright © 2012 European Society of Radiology