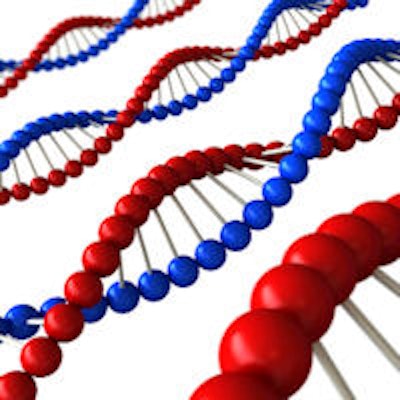
Medical physicists are responsible for technical aspects of radiotherapy delivery, by ensuring the quality assurance (QA) of the equipment delivering radiation, and of the radiation plans that define the patient's treatment. But is this the only role of the medical physicist today? How much can the profession contribute to research? Do medical physicists need to broaden their perspective?
These questions were the subject of a scientific session at the 2013 American Association of Physicists in Medicine (AAPM) annual meeting, held in Indianapolis, Indiana. Three medical physicists talked about their perspectives on the involvement of their profession in research.
Knowledge engineering
Today's generation of medical physicists have the opportunity to transform the way that individual cancer patients are treated through the adoption of knowledge engineering in daily clinical practice. This concept, also referred to as "rapid learning healthcare," is based on the principle of using and reusing existing data to constantly improve decision-making and treatment outcome.
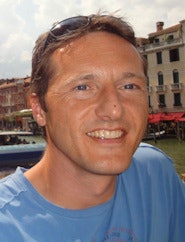
For Erik Roelofs, medical physicist and program manager and PhD candidate of MAASTRO Clinic's Knowledge Engineering research group in the Netherlands, a primary objective is to create clinical decision tools and prediction models from data acquired from numerous cancer treatment centers. André Dekker, who heads this group, is in the process of getting cancer treatment centers in Australia to contribute data and join centers from Italy, Germany, China, the U.S., Belgium, and the Netherlands in this effort, known as EuroCAT.
This is now becoming possible through the adoption of electronic patient medical records by many countries throughout the world. Medical professionals have diligently recorded medical data for decades. But unlike industries such as banking that routinely and transparently share information electronically on a global scale, the medical profession has lagged behind. Only within the past decade have electronic patient records proliferated, and many challenges exist to make these data transparently interchangeable in a usable form for data mining.
"Clinical trials currently provide the data of outcomes used to decide cancer treatments. However, patients enrolled in clinical trials represent only about 3% to 5% of cancer patients. So the chances are very high that when an oncologist or radiation oncologist decides upon a course of treatment for an individual patient based on the results of a clinical trial, the patient may not have the same disease characteristics as the clinical trial patient cohort," Roelofs explained. "What this means is that the patient may not have the ideal personalized treatment."
By being able to acquire data from numerous sources, and analyzing it through automated data mining, it will be possible to develop prediction tools, and from that, clinical decision support software designed for optimized individualized patient treatment. The current method of obtaining evidence -- namely through clinical trials -- is too costly and unpredictable. If collected, data that are routinely gathered through patient care and clinical research can feed an ever-growing set of coordinated databases. Information-rich, patient-focused data can transform subsequent care delivery, Roelofs emphasized.
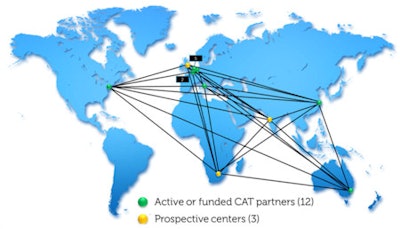
Dekker and his team at MAASTRO Clinic have been working on this. They have been developing tools designed to overcome the challenges of scattered and unstructured data, written in multiple languages, to be used for rapid, informed decision-making.
The group is also involved with is the ROCOCO (Radiation Oncology Collaborative Comparison) trial. This international, multicentric in silico clinical trial in lung, prostate, and head-and-neck cancer is investigating whether with the same dose on the tumor, particle therapy is cost-effective in comparison with photon treatment modalities like conventional radiotherapy and intensity-modulated radiotherapy (IMRT).
"How does a radiation oncologist determine whether proton therapy is better and more effective for an individual patient than photon therapy?" Roelofs asked rhetorically. "Prediction models are needed, which then must be continuously re-evaluated on different patient datasets from different regions to ensure its population-specific strength. We strongly believe in the open-source, open-data concept, and as such offer our prediction models and image data via the PredictCancer and CancerData platforms."
The next step for Roelofs is to bring these together in a decision support system for protons. "By combining the MISTIR [multicentric in silico trials in radiotherapy] platform with dosimetric comparisons from ROCOCO, and the prediction models from PredictCancer with state-of-the-art normal tissue complication prediction models, and offer this via a new Web-based platform called PRODECIS, we can quickly inform referring specialists whether patients are eligible for proton therapy or not," he added.
"Working as such in the center of medical physics, medical informatics and more recently, knowledge engineering is a typical job for a medical physicist. By orchestrating the joint efforts from these complimentary disciplines, with data and knowledge being shared in a standardized, instant, and global manner, we can create validated decision-support systems with a focus to optimally integrate them in the clinic," Roelofs concluded.
Nontraditional collaborations
Thomas G. Purdie, a medical physicist at the Princess Margaret Cancer Center in Toronto, Ontario, believes the time is right for medical physicists to interact with professionals in the fields of engineering, computer science, and informatics. These are nontraditional research domains, but ones that offer great potential opportunity for skilled professionals to collaborate and create new innovations.
The Princess Margaret Cancer Center is affiliated with the University of Toronto. As a member of the university's department of radiation oncology, Purdie says it is easy to talk with potential collaborators. He described one project automating QA processes that greatly benefited from collaboration with computer scientists and industrial engineers.
Another project, the development of an automated technique for two-field tangential whole breast IMRT planning, created software that has become a commercial product. Purdie developed an automated algorithm that is now integrated into RaySearch Laboratories' RayStation clinical treatment planning system. The algorithm emulates all treatment planning steps and decisions currently performed by a radiotherapy planner, including organ segmentation, beam optimization, IMRT optimization, and dose calculation, tasks that can be highly time-consuming to perform manually.
The software, which enables dosimetrists to produce complex clinical treatment plans and automated QA reporting in less than 10 minutes, is the standard of care for tangential breast treatments at Princess Margaret and has been used to treat more than 2,000 patients since 2009. The software was licensed to RaySearch in September 2012.
"This tangible success has made it easier to initiate collaborative processes with engineers in different disciplines and computer scientists. Medical physicists do not typically have a strong background in these fields, but their ideas for automation make collaboration a natural fit," Purdie said. "I do clinical work like every other medical physicist, but I had an idea that could make a process better, and I'm delighted that by working with peers outside my discipline, this turned into a product that everyone could use."
Purdie also noted that clinical research proposals for grants that involve multidisciplinary collaboration tend to have a higher chance of funding. And engineers or computer scientists find it easier to get grants when there is a clinical application. "It broadens the scope for everybody," he said. "Ideally our work will help automate and therefore improve the work of radiation oncologists and treatments for cancer patients."
Foundational research: Ask bigger questions!
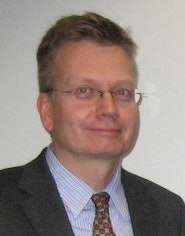
Thomas Bortfeld, a professor of radiation oncology at Harvard Medical School and director of the medical physics division in radiation oncology at Massachusetts General Hospital in Boston, Massachusetts, expressed concern that many of his peers in the U.S. do not have the time they need to conduct fundamental "foundational" research. He defined foundational research as research with the potential to open entirely new directions in medical physics with long-term impact, such as the research that led to CT and IMRT.
Bortfeld illustrated his concern by categorizing the most highly scored research presented at this year's AAPM meeting, including the "best in physics" abstracts and the young and junior investigator awards, into four categories ranging from foundational research to clinical implementation. It turned out that none of the 26 most lauded abstracts fell into the foundational research category; the vast majority described technological improvements.
The problem with this trend is that the clinical innovations of tomorrow rely on foundational research of today. Without the fundamental research to "fuel" future innovation, the field is at serious risk of drying out.
"The role of the medical physicist as we know it may become extinct," Bortfeld warned. "The trend is further accelerated in the current era of cost-cutting and economic pressures. Medical physicists working in both academic and nonacademic hospitals have to deal with workloads that consume their time and prevent them more and more from undertaking research, let alone foundational research. In the near future, medical physicists may be forced to focus primarily on workflow, efficiency, and automation. As Jens Overgaard (from the Institute for Klinisk Medicine in Aarhus, Denmark) put it at the 2013 ESTRO conference: 'The fun for medical physicists is over, now there is hard work ahead'."
So is it possible to break out of this vicious circle? Bortfeld thinks that while some re-focus on cost-efficiency and workflow is unavoidable, and probably healthy, one cannot forego foundational research. He encourages medical physicists to ask bigger questions, not those that lead to small incremental improvements, but questions that trigger foundational research with potentially much bigger impact.
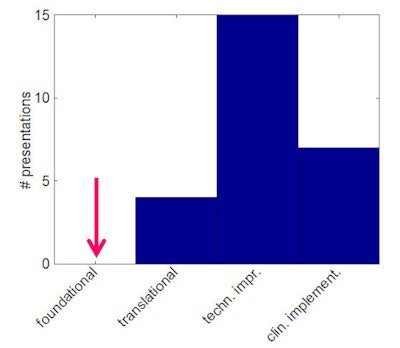
Those questions do not need to be answerable by individuals or small groups, certainly not within a short time frame. Instead, they could be answered in collaboration with physicists and physical scientists in other areas, such as those in the NCI-funded Physical Sciences Oncology Centers (PSOC). A workshop to be held in November on "Expanding Horizons" between AAPM and PSOC will discuss some of those bigger questions.
© IOP Publishing Limited. Republished with permission from medicalphysicsweb, a community website covering fundamental research and emerging technologies in medical imaging and radiation therapy.