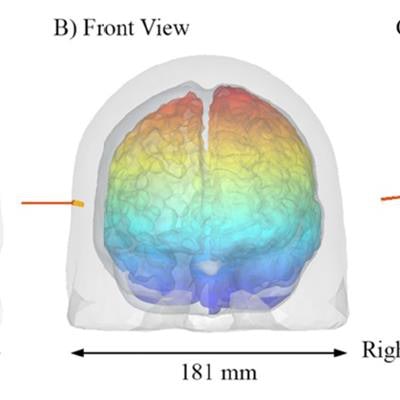
Neurodegenerative disorders such as Alzheimer's disease are often associated with brain atrophy. During disease progression, the brain loses neuronal tissue and reduces in size inside the skull. The left-over space then fills with additional cerebral spinal fluid (CSF) -- making CSF variation a potential biomarker for the presence of Alzheimer's disease.
Currently, visualizing such structural changes is achieved using MRI or CT, neither of which are suitable for time-resolved monitoring, due to high costs or the risks of repeat exposure to ionizing radiation. Instead, a research team from Greece and Italy has proposed the use of noninvasive time-resolved near-infrared (tr-NIR) measurements to track brain atrophy during disease progression (Biomedical Optics Express, 1 September 2018, Vol. 9:9, pp. 4094-4112).
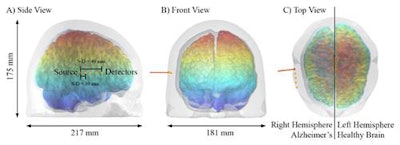
"While MRI and CT excel in term of resolution, if we aim to extract only one specific feature we could rely on more efficient imaging techniques," explained first author Daniele Ancora from the Institute of Electronic Structure & Laser at FORTH. "Tr-NIR imaging is fast, cheap, and harmless. After positioning a NIRS head-cap with sources and detectors, data acquisition would be near-instantaneous, enabling measurements of a wide range of patients with very little economic and resource effort."
Computational models
Ancora and colleagues employed a computational approach to study the potential of tr-NIR for visualizing atrophy progression. They generated two brain structure models -- a simple cylindrical model and a more complex human-head atlas -- and used Monte Carlo photon propagation to simulate the results of a longitudinal experiment.
The simple models constituted 100-cm diameter cylindrical mesh volumes, with layers representing skin and skull, CSF, gray matter, and white matter. In each mesh, the skin and skull and gray matter tissues had constant thickness, the CSF size increased to mimic Alzheimer's progression, and the white matter tissue decreased accordingly. The researchers examined seven CSF thicknesses, from 0.0 mm to 15.0 mm. They placed time-resolved photon detectors on the skin and skull surface to create concentric detector ring arrays and simulated a pencil-beam source incident upon the center of each mesh.
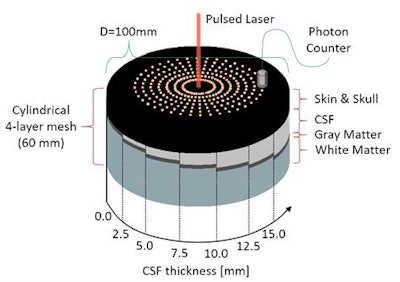
For the head model, the team started with the human-head mesh structure Colin27 and used meshing tools to implement an erosion algorithm that reproduced brain shrinkage. They built 11 meshes: stage 1 to 10 of disease progression and stage 0 for the original Colin27 model. They positioned a pulsed beam source perpendicular to the skin and skull surface on the right hemisphere and placed four time-resolved photon detectors next to it.
The researchers used the mesh-based Monte Carlo tool to resolve photon diffusion within the models. To account for variation in tissue optical properties among individuals or disease stages, they employed two different CSF scattering coefficients: considering CSF as a nearly transparent nonscattering layer; and having more turbid optical properties.
To simulate a realistic measuring system, the team experimentally acquired the instrument response function (IRF) of a typical fNIRS detector. They convoluted this measured IRF with the simulated photon distributions to estimate its impact.
DTOF distributions
The team first assessed the distribution of time-of-flight (DTOF), which carries information about photon diffusion, for the seven cylindrical models. At early detection times, increasing CSF thickness did not result in significant variation in DTOF. This is expected, because early photons spend most of their time in the superficial skin and skull layer and not in deeper tissue regions.
At later photon gating times (greater than 2,500 ps), however, photons may have traveled through the skin and skull into the CSF layer. Here, brain shrinkage visibly changed the slope of the detector response, with higher CSF thicknesses corresponding to less steep slopes. The researchers observed a steep slope change during the initial increase in CSF thickness, suggesting higher sensitivity at early stages of brain atrophy. The change in slope with CSF thickness was not exactly the same for the two scattering coefficients, but the overall trend was preserved.
Results for the human head model were comparable, with increasing CSF thickness flattening the slope of the DTOF at late gating times. For both models, convolution with the IRF did not affect the slope of the late-timing DTOF curve, suggesting that CSF variations can be measured using a real instrument.
The modeled detector response was also affected by the scattering coefficient, with increased CSF scattering flattening the slope in a similar manner to the CSF thickening. "In a hypothetical scenario in which both parameters vary, we would still be able to see an effect on the response curve," Ancora explained. "A change in CSF optical parameters might actually be another indicator of disease progression, but to assess that we need more investigations, such as spectroscopy."
Ancora notes that tr-NIR spectroscopy is available in almost all tr-NIR systems and could enable simultaneous acquisition of response functions over whole visible-NIR spectrum. "The optical turbidity of the CSF might vary differently in separate spectral windows, giving us another tool for evaluating possible variations connected with disease," he said.
The next step will be to verify the computational results with experimental evidence. "For this, we could make use of the existing tr-NIRS facilities at Politecnico di Milano," Ancora told Physics World. "If the experiments match our numerical predictions, we will consider to taking this forward in a real clinical scenario."
© IOP Publishing Limited. Republished with permission from Physics World, a website that helps scientists working in academic and industrial research stay up to date with the latest breakthroughs in physics and interdisciplinary science.