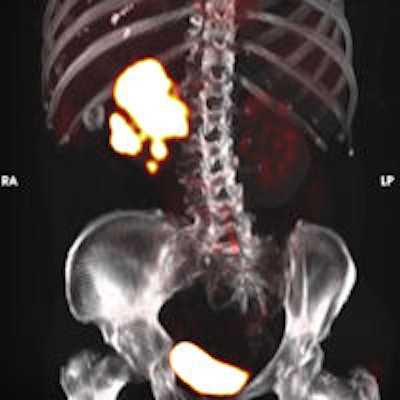
Detectors for use in time-of-flight (TOF) PET must offer high detection efficiency, good spatial and energy resolution, and excellent timing resolution, as well as provide depth of interaction information. A promising candidate that meets all of these requirements is the monolithic scintillator detector, used in combination with a digital silicon photomultiplier array.
Monolithic scintillators estimate gamma photon interaction points on the basis of measured light distributions. As the scintillation light is distributed over a large number of photosensor pixels, this results in a low light intensity per pixel. Therefore, any photosensor used with this detector must offer extremely low readout noise, such as that exhibited by a digital silicon photomultiplier (dSiPM).
"It appears that the use of dSiPM arrays for the readout of monolithic scintillators enables unprecedented performance, especially in thicker crystals -- of roughly 20 mm -- which are required for clinical PET," said Dennis Schaart, PhD, from Delft University of Technology in the Netherlands. To investigate the potential of this combination, Schaart and colleagues characterized a monolithic crystal detector read out by a prototype dSiPM array (Physics in Medicine and Biology, 7 May 2013, Vol. 58, pp. 3061-3074).
Crystal characterization
The team studied a 24 x 24 x 10-mm 0.2% Ca co-doped LSO:Ce scintillator. The dSiPM (developed by Philips Digital Photon Counting) comprised a 4 x 4 array of silicon dies, each containing 4 dSiPM pixels. The pixels in each die share a common controller and time-to-digital converter, resulting in a single time stamp per die for each event. The base surface of the crystal was coupled to 3 x 3 dies.
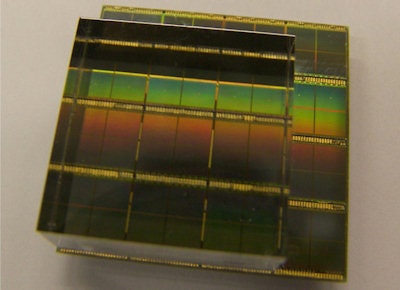
To assess its performance, the researchers employed a paired-collimator setup to irradiate the detector at well-defined positions with 511 keV annihilation photons. Coincidence events were recorded for 50 seconds at each grid position.
The researchers only processed scintillation events in which at least eight of the nine dies covered by the crystal were read out. Dies were not read out if the number of impinging photons was too small to meet the trigger criterion. The authors note that the next-generation dSiPM array may implement new trigger logic to overcome this issue.
Spatial resolution
To assess the detector's accuracy in determining photon interaction positions, Schaart and colleagues created detector point spread functions (PSFs) based on differences between estimated coordinates and their respective "true" irradiation points. Full-width-at-half-maximum (FWHM) values of cross-sections through the PSFs were used as measures of the spatial resolution.
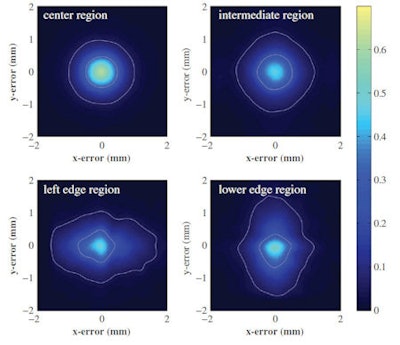
Previous studies have shown that the spatial resolution of monolithic scintillator detectors can vary with the interaction position, thus the researchers constructed separate PSFs for a center region, four edge regions, and an intermediate region. Averaged over the entire detector, the device exhibited a spatial resolution of 1 mm FWHM. This varied from just below 1 mm FWHM in the center region (in both x and y directions) to about 1.2 mm FWHM for interaction positions near the crystal edges.
The team also calculated a bias vector at each reference beam position, to evaluate any possible bias in position estimation. The magnitude of the bias was negligible (less than 0.1 mm) in the detector center, remaining well below 1 mm even close to the corners of the crystal.
MLITE method
Another crucial ingredient for efficient TOF PET is excellent timing resolution. So the researchers have developed a maximum likelihood interaction time estimation (MLITE) algorithm to derive the time of interaction from the multiple time stamps obtained for each scintillation event.
One problem in the improvement of TOF performance, Schaart said, is the position-of-interaction-dependent time taken for scintillation photons to travel through the crystal and reach the photosensor, which imposes a limit on the achievable timing resolution. In crystals of sufficient thickness for clinical PET (roughly 20 mm) this phenomenon makes it difficult to achieve a coincidence resolving time (CRT) of less than 200 ps FWHM.
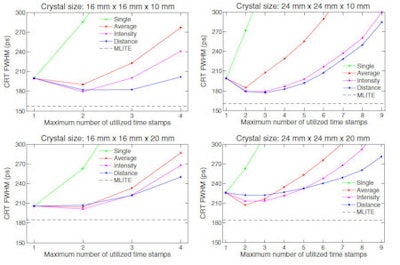
MLITE can at least partially correct for this effect. In results published in a companion paper, Schaart and colleagues demonstrated CRTs of 157, 185, 161, and 184 ps FWHM using MLITE for pairs of monolithic scintillator detectors with dimensions of 16 x 16 x 10 mm, 16 x 16 x 20 mm, 24 x 24 x 10 mm, and 24 x 24 x 20 mm, respectively (Phys Med Biol, 7 May 2013, Vol. 58, pp. 3243-3257).
"The monolithic scintillator, the dSiPM array, and clever signal processing together make it possible to meet all of the requirements for next-generation clinical TOF-PET/CT and TOF-PET/MR scanners: sub-200 ps CRT, sub-2 mm spatial resolution, depth-of-interaction capability, good energy resolution and, last but not least, high sensitivity," Schaart said.
Next, the TU Delft team hopes to demonstrate that the monolithic scintillator is a viable option for building a clinical scanner. "Amongst others, this requires the development of a practical calibration method for a full ring of monolithic scintillator detectors, a topic that we are already working on," they said.
Schaart points out that the detector's simple construction -- a monolithic crystal with a single dSiPM coupled to its back surface -- and its fully digital implementation make it highly scalable. "It should be feasible to reproduce the high performance in a large number of detectors, necessary to build a full clinical scanner, at acceptable costs," he told medicalphysicsweb.
Editor's note: The PET/CT image on the home page is courtesy of Dr. Osman Ratib, PhD, Geneva University Hospital in Switzerland.
© IOP Publishing Limited. Republished with permission from medicalphysicsweb, a community website covering fundamental research and emerging technologies in medical imaging and radiation therapy.