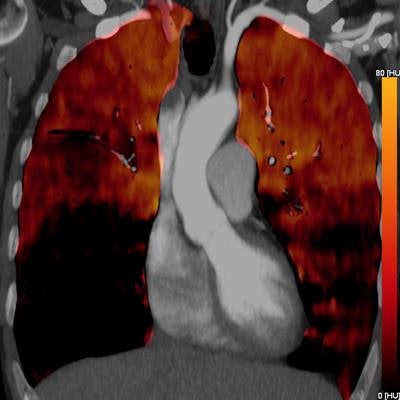
In the right hands, dual-energy CT (DECT) for acute pulmonary embolism (PE) can help boost sensitivity and detection, especially for endoluminal clots in small segmental or subsegmental lung vessels. That's the view of German researchers, who've given a master class on the topic.
Pulmonary blood volume (PBV) images representing iodine concentration allow for visualization of perfusion defects due to obstructing clots, though nonobstructing clots are not necessarily associated with perfusion defects, according to Dr. Aleksander Kosmala and colleagues at the Institute for Diagnostic and Interventional Radiology, University Hospital Würzburg.
Using a three-material decomposition algorithm (air, soft tissue, and iodine), PBV maps based on the detection of iodine in pulmonary parenchyma show the iodine concentration in lung parenchyma during a study, but because only one time point is measured, PBV maps are only a surrogate measure for real perfusion (which would require repeated measurements at multiple points).
"PBV defects may be due to pulmonary embolism and other causes, such as emphysema, beam-hardening artifacts, motion artifacts, or segmentation-threshold transgression," they explained in a digital poster presentation at RSNA 2019. "Therefore, PBV images should always be read additionally to regular CT pulmonary angiography images."
Blood clots that do not lead to a total vessel obstruction are not necessarily associated with a perfusion defect on PBV images (see figure below). On the other hand, completely obstructing blood clots normally lead to corresponding perfusion defects.
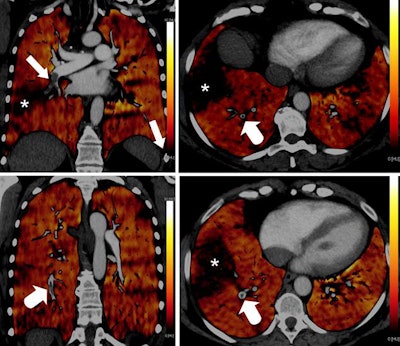
Dual-energy CT scans involve the acquisition of two datasets at low and high kilovoltages, as follows:
- Dual-source DECT (e.g., Siemens Somatom Force): Two pairs of x-ray tube/detector systems 95° apart rotate simultaneously around the patient. Dose-efficient, good spectral separation, but limited field-of-view (FOV).
- Dual-detector layer DECT (e.g., Philips IQon): Photon energy spectrum separation using a special detector system. DE information is always available, but limited spectral separation.
- Rapid voltage switching DECT (e.g., GE GSI Xtream): Tube voltage alternates between a low and high kV value. Full FOV, but possible radiation dose increase.
- Dual-spin DECT (e.g., Canon Aquilion One): Sequential acquisition at different tube voltages (as subsequent helical scans or stepwise sequence), but high risk of misregistration.
- Twin-beam DECT (e.g., Siemens Somatom Edge): Prefiltration of the x-ray beam with a tin/gold filter results in a high/low energy spectrum, but possibly limited spectral separation.
Depending on the contrast injection protocol, differences in vessel attenuation and pulmonary iodine concentration can be observed. High volumes of contrast medium (e.g., ≥ 60 mL) injected at a high flow rate (e.g., ≥ 4 mL/sec) usually result in superior vessel attenuation and homogenous high parenchymal iodine concentration. Lower volumes of contrast medium may result in only moderate vessel attenuation and patchy, inferior parenchymal iodine concentration, they added.
Emphysema causes a true decrease in pulmonary circulation due to lung parenchyma destruction. This is visualized in PBV images as a perfusion defect, but it should not be mistaken for hypoperfusion due to embolism (see figure below).
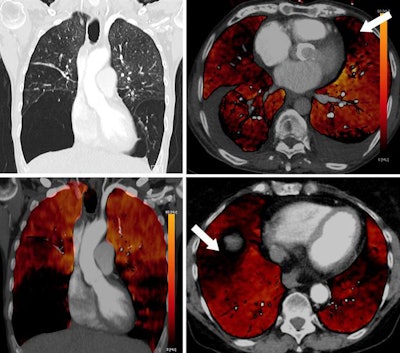
An algorithm allocates a ratio of air and soft tissue within a voxel, determining lung parenchyma containing air, and iodine content is quantified and visualized in color-coded perfusion maps.
"Monoenergetic reconstructions at lower kV levels possess an intrinsically higher vessel contrast, leading to improved diagnostic confidence in small vessels and in examinations with inferior vessel contrast due to suboptimal contrast bolus timing," the authors noted.
Dose neutral DECT of the chest is feasible at similar image quality and image noise compared to standard 120-kV protocols, and DECT of the chest for acute PE can even result in reduced radiation dose compared to a single-energy protocol on the same scanner and a 120-kV protocol on a conventional 64-slice CT scanner, at comparable subjective and objective image quality, they continued.
Dual-energy acquisition generates different image datasets: low-kV and high-kV images from the respective tubes, and a weighted average image composed of data from both tubes in a variable proportion (e.g., 60% low kV + 40% high kV @ 90/150-Sn kV) comparable to a regular 120-kV image. Further dual-energy specific postprocessing requires dedicated software.
Possible pitfalls
Kosmala and colleagues said it's important to keep aware of these potential pitfalls and limitations:
- Hyperdense contrast material in the vessels may cause beam-hardening artifacts, typically adjacent to the subclavian vein, brachiocephalic vein, or superior vena cava, leading to a misregistration on the PBV map. Metallic implants, such as a breast expander or a spinal fixateur, can also cause beam-hardening artifacts.
- The threshold function used by the three-material decomposition algorithm evaluates the iodine concentration only in voxels in the range between -960 HU and -600 HU, when set to default values. Voxels with HU values exceeding these thresholds are excluded from the calculation and displayed as PBV defects.
- Increasing the threshold range can be used to visualize iodine concentration in tissues with lower or higher attenuation, e.g., areas of ground-glass opacity.
- In scanners using the dual-source, dual-energy technique, the high-kV detector covers a FOV of 50 cm, while the low-kV detector is limited to a FOV of 26 cm to 35 cm, depending on the scanner generation.