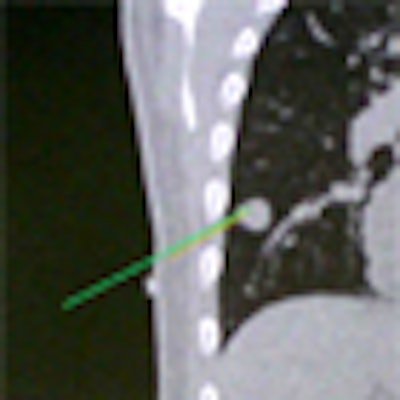
The interventional radiology suite of tomorrow is already developed and ready to go -- notwithstanding the minor detail of clinical experience -- with robust systems capable of working with humans to perform any number of tasks more accurately than traditional methods, and often with less radiation exposure.
In a talk this summer at the 2011 Computer Assisted Radiology and Surgery (CARS) conference in Berlin, Kevin Cleary, PhD, said clinical experience is the magic ingredient that can help move these technologies quickly from "bench to bedside," and into clinical practice.
"A lot of this technology is available now and developing rapidly, so the key is for scientists and clinicians to work together for better patient care -- I think that's the real challenge for us nowadays," said Cleary, who is technical director of the bioengineering initiative at Children's National Medical Center in Washington, DC. "We want to enable rapid prototyping so we can transition to clinical care as quickly as possible. When you go to the clinical world you find all kinds of problems that didn't show up using your phantoms and other studies."
The interventional radiology suite includes several tools that enable navigation in image-guided procedures, as well as tool kits to facilitate vertebroplasty, bronchoscopy, radiofrequency ablation (RFA), and robotics, which can be used to perform spinal nerve blocks and lung biopsies, among other procedures, Cleary said. For following the procedures in real-time, electromagnetic tracking represents a step forward from optical tracking in that it allows visualization of the tracking device inside the body, he said.
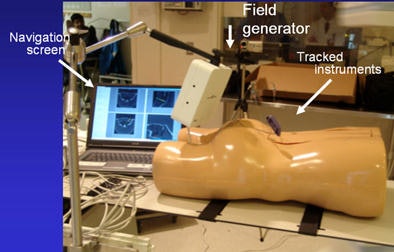
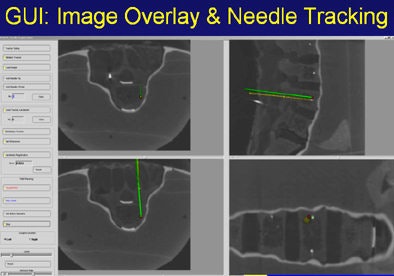
"You put small sensors on the tip of your needle and you have a small field generator that generates a time-bearing magnetic field that pulses it in x, y, and z directions," Cleary said. "The pulsation from the generator induces a voltage stream in the sensor coil that can be read back by a computer and identify where an object is located in space."
Nevertheless, traditional optical tracking remains the most common and familiar type of tracking system in the interventional setting.
"It's line-of-sight, it's very accurate, but you can't track inside the body," he said. "What electromagnetic tracking allowed us to do was track inside the body, and now we have the chance to use image-guided systems for abdominal intervention -- and it opens up a whole new field for this kind of surgery. We can build a system where we now have a field generator, a sensor coil with needle, track the needle, and give an image overlay on a four-quadrant display in all these systems" (Aurora electromagnetic tracking, Northern Digital) Cleary said. The graphic user interface shows the planned path is in green and the virtual overlay in yellow.
Instead of looking back and forth and checking with the scanner as you advance the needle, you can watch the virtual reality display and put your needle down, he noted. When the image seems right, the interventionalist goes back for a confirming x-ray image; the procedure can then be performed with conebeam CT -- which in a sense has brought CT to the interventional suite.
Conebeam CT is another key interventional tool that is increasingly being brought to the operating room in hybrid interventional procedure rooms; systems such as the Zeego angiography system (DynaCT, Siemens Healthcare) work in tandem, featuring a C-arm x-ray source on one end and a flat-panel detector on the other. "You can rotate the C-arm more than 180°, generating multiple oblique images," Cleary said. "It's becoming more common in the interventional suite and now in some operating rooms. GE Healthcare, and Philips Healthcare also offer conebeam systems, he said.
Cleary and colleagues performed a clinical trial to demonstrate the accuracy of automated lung biopsy in the CT suite. The procedure begins with a CT scan, or conebeam CT in the interventional radiology suite, and is followed by a registration step that is required to allow the clinician to locate the fiducial markers first in CT space, then electromagnetically, he said.
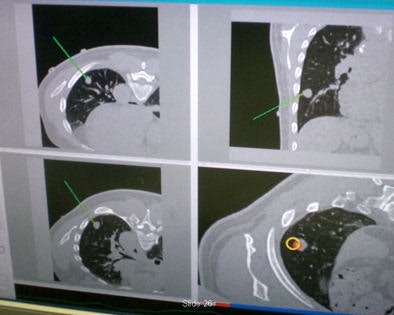
"Touch [the marker images] with an electromagnetically tracked sensor and you know where they are in electromagnetic space," he said. "Registration and image overlay allows respiratory tracking during the procedure. You can navigate off the display and get a confirming image on completion."
Four patients participated in the study. In three patients needle was placed correctly based on the image-guided system. In the fourth patient, the needle was off-target but close, and the position was corrected using the standard technique, Cleary said.
RFA
Image-guided radiofrequency volumetric treatment planning for radiofrequency ablation of tumors is also being performed with a conebeam CT and C-arm system. RFA allows large tumors to be treated with overlapping spherical zones. The minimally invasive cancer treatment works by inserting the probe into the tissue, and "you literally heat up and cook the tissue and sometimes cover the whole tumor and you have to do multiple whole tumors," Cleary said.
A key benefit of the system is that it addresses one of the most significant challenges facing RFA: successfully targeting the tumor and providing adequate ablation coverage of it, Cleary explained. An improperly placed probe not only provides incomplete tumor ablation, but could also damage nearby delicate tissues or organs. Despite the availability of larger electrodes, many procedures require multiple ablations to maintain a 1-cm margin. Multiple ablations are difficult to execute as there is no way to visualize the overlapping areas or to distinguish ablated areas from nonablated areas.
Standard techniques offer no way to visualize the overlapping areas, and due to the lack of any real-time image guidance, repeated insertions and positioning of the electrode may be required to hit the target lesion and establish sufficient margins, Cleary said.
Incomplete ablation is more than a technical problem, because unablated tissue containing residual tumor can result in tumor recurrence, he said. Residual tumor often grows in scattered, nodular, or eccentric patterns, making it difficult to treat after an initial ablation. Studies have shown that complete necrosis of the tumor was achieved in less than half of lesions larger than 3 cm.
"We can give you image guidance and navigation and actually help you carry out these procedures: an image-guided system based on electromagnetic tracking that provides improved visualization and probe placement, allowing clinicians to more thoroughly ablate the tumor while sparing healthy tissue," Cleary said. "We have a preprocedure planning and intraprocedure component [for guidance during the procedure] to carry that out."
For ablating liver tumors, the patient is prepared by placing four separate electromagnetically tracked skin patches above the liver. Contrast-enhanced CT images are obtained from the lung base through the abdomen and reconstructed to 1.0 mm. The relevant anatomy, including the tumor and a 1-cm margin, is segmented. Treatment planning determines the overlapping probe placement. The tracking probe is positioned near the patient and enabled. Image registration is performed using paired-point matching. The system then provides the physician with targeting assistance and image overlay so the treatment plan can be carried out.
Powered by open source
All of the image-guided treatment models are made possible by the use of open-source software, Cleary said. "You should use an open-source package because a lot of people spent a lot of time developing this and you don't want to reinvent the wheel," he said. Various academic centers (including his own former employer, Georgetown University in Washington, DC) have created a series of tool kits that can be adapted to just about any interventional need including robotic arm movement, tractors, navigation systems, and more. The main originating centers are:
- MARVIN - University of Bern, Switzerland
- CISST - Engineering research center, Johns Hopkins University, Baltimore
- MITK - Medical and bioinformatics, German Cancer Research Center, Heidelberg
- OpenMAF - Institute of Orthopedic Research, Bologna, Italy
- IGSTK - Isis, Georgetown University, Washington, DC
- Slicer-IGT - Brigham and Women's Hospital, Boston
Clinical implementation can be speeded up significantly with the adaptation of open-source tools, Cleary said. "We actually want to enable rapid prototyping of the actual development so we can transition to clinical care as quickly as possible," he said. "When you go to the clinical world you find all kinds of problems that didn't show up using your phantoms and other studies."
Medical Robotics
Cleary's Georgetown University interventional suite uses Zeco, a joystick-controlled medical robot suite. Physical manipulation of a touchscreen changes the orientation of the robot, whose applications include lung biopsy via fluoroscopy.
As the targeted lung moves in and out the scan plate is kept stable by coordinating the robotic system to the scanner. John Hopkins University's Dan Stoianovici, PhD, and the National Institute of Health's Brad Wood, PhD, designed and built a rotating needle driver for the robotic system that spins the needle to reduce friction, Cleary said.
Sheng Xu from Baltimore's Johns Hopkins University, along with Cleary and Dr. Charles White from the University of Maryland, performed robotically assisted lung biopsy under CT fluoroscopy, first in phantoms, and then successfully in a pig, Cleary said.
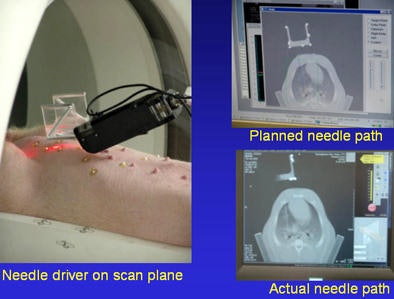
Spinal nerve blocks are also done with robotics. Cleary and colleagues performed a study to show that a physician-controlled robotic needle driver is equivalent in safety and effectiveness to the standard manual technique for needle placement in nerve and facet blocks in the perispinal region.
A key feature of the needle driver is release of the needle upon contact with the skin, he said. "One of the problems with the robot holding the needle steady in the body is that when the patient starts to move or breathe again you can tear up the tissue -- so you have to be able to release the needle at a preprogrammed force level."
"The take-home message is that a lot of this technology already exists," Cleary said. I think now the talks should be on how we bring the technology into the clinic. We all talk about this but it's harder than it looks. We need pilot clinical trials and more open-source software to facilitate the transition."