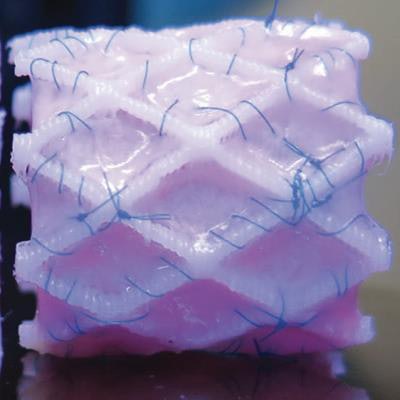
A new 3D-printed polymer stent overcomes many of the limitations of conventional nitinol stents for use with tissue-engineered heart valves, offering the potential of use in children, according to an article in 3D Printing and Additive Manufacturing.
Several development trends have paved the way toward this new kind of cardiac stent, for which technology is aligning with the need for minimally invasive implantation techniques for decellularized tissue-engineered heart valves (DTEHV), according to a Dutch study team.
The proof-of-concept study from Eindhoven University of Technology in the Netherlands describes the design and manufacture of a polymer stent with mechanical performance properties similar to that of conventional nitinol stents that have been used for heart valve implantation in animal trials.
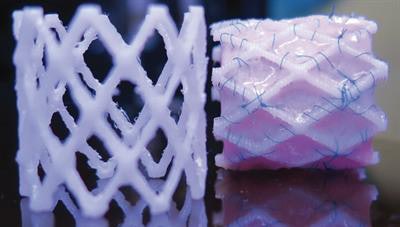
The investigators created the computer-designed stents from a commercially available 3D polymer, conducted crush and crimping tests to validate the results predicted by the computational model, and used accelerated hydrolysis to assess degradability in the human body.
Computational-based 3D-printed self-expandable and biodegradable polymer stents can be successfully designed, concluded Drs. María Sol Cabrera, Bart Sanders, and colleagues at Eindhoven.
"By adopting 3D printing as a manufacturing method, we were able to evaluate the crimpability and self-expandability of our stent designs in the lab just a few minutes after completing our simulations," Cabrera wrote in an email. "This allowed us to demonstrate that with a rational computational design, bioabsorbable polymers can be applied to produce strong stents that can ultimately replace the use of metals stents in pediatrics."
About 280,000 patients undergo heart valve surgery every year, growing annually to an expected 1 million by 2050, the authors wrote. New DTEHV developments are promising in their capacity to remodel, based on sheep studies, which revealed rapid host cell repopulation and extracellular matrix production, two indicators of stent growth potential. Advances in valve development go hand in hand with the need for minimally invasive stent implantation, the team wrote (3D Printing and Additive Manufacturing, March 2017, Vol. 4:1, pp. 19-29).
Limitations of current metal stent technology include a lack of growth capacity, which can lead to long-term complications such as hyperplasia. And bioabsorbable 3D-printed stents would enable clinicians to avoid another round of surgery to remove the implants after the new valve has integrated itself into the heart tissue.
Unfinished work
The new polymer stent still lacks a key feature, however. If DTEHVs are to be used in children, they must be able to accommodate growth, which will require the development of new printing materials.
"The premise behind this opportunity is to eliminate the need for redo surgeries, to further expand the metal stent as the patient grows, and replace it with structures that disappear by themselves after doing their job," Cabrera told AuntMinnieEurope.com.
Polymers have several other potential benefits, including a low rate of late thrombus formation, and less interference with MRI than metal stents, according to the authors, who are aiming for equivalent performance to nitinol stents used with valve implantation in sheep.
A nitinol stent was used to design the polymer stent based on fused deposition modeling (FDM) technology combined with a commercially available thermoplastic copolyester elastomer (TPC). The polymer stent was composed of a repeating design with three rings of 40 struts, connected by tilted bridges.
Tensile tests were used to mechanically characterize the TPC and used as input for the computational model. The authors tested FlexiFil (Formfutura), a flexible TPC filament used for FDM applications, and 3D-printed dog bone samples from the same material to assess the production procedure.
Design optimization
The optimization procedure is an iterative process that adjusts the polymer stent geometry to 1) allow crimpability of the stent to a diameter of 12 mm, and 2), obtain a [radial force] RF comparable to that of nitinol stents, Cabrera and colleagues explained. Parameters including width, thickness, and number of struts can be adjusted to modify the response of the polymer stent.
Based on the computationally engineered designs, 3D-printed stents were created and subjected to crush, crimping, and self-expansion tests for mechanical validation using FE software (ABAQUS 6.14, Dassault Systèmes Simulia).
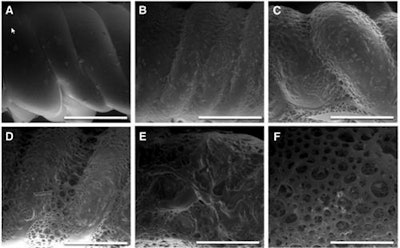
An in vitro degradation test of the polymer stents was performed to assess the biodegradability of the TPC via hydrolysis. Additional mechanical tensile tests were used to assess degradation over time under conditions created to mimic conditions in the body. The authors measured the effects of hydrolysis on the polymer's molecular weight via gel permeation chromatography (GPC), while stents were examined using scanning microscopy, the study team reported.
Assessing the influence of 3D printing on the mechanical properties of the stent required comparing stress-strain curves obtained from the polymer wires with the 3D-printed dog bone samples. The printed samples deviated from the linear behavior with lower stress values than the wires, however, the decay in tensile properties was not extreme, the team wrote.
Adjusting the design parameters to enhance crimpability and RF simultaneously requires a delicate balance between opposing effects, the authors explained. Increasing the width of the struts increases the RF of the stent, but reduces crimpability and increases plastic deformation during crimping, leading to small diameters after expansion. Increasing width also requires reducing the number of struts.
The design delivering the closest performance to a nitinol stent of similar diameter consisted of 24 struts, six rows of struts, an outer diameter of 30.5 mm, and a length of 30 mm, the authors wrote. The prototyped stent differed slightly from the computationally derived geometry, necessitating the creation of a strut sketch from a digital image of the stent prototype, which was incorporated into the simulations.
To simulate crushing, the group compared experimental force with displacement curves resulting from the crush tests of the polymer 3D-printed prototypes, finding good agreement with the computational models. Crimp tests showed a plastic deformation repetitive pattern that is more pronounced at the zones where the two struts meet, reducing stent diameter even after load removal.
Gel permeation chromatography showed the polymer degrades over time, as revealed in smaller molecular weights. Mechanical analysis showing a decay in the maximal applicable stress over time, falling to half of the initial force after 10 days of enhanced in vitro degradation. Electron microscopy showed an increase in surface roughness increased over time, and an increase in porosity.
The bottleneck of the otherwise successful project is the lack of materials with sufficient mechanical properties to meet the design needs.
"To move forward with this approach, it is not only about finding biocompatible materials with the right balance between strength and degradation force," Cabrera said. "Once you do, you need to process this material appropriately to shape the stent struts. Therefore, the choice of 3D printing technique also plays an important role that may add more requirements to your material."
For example, traditional bioabsorbable polymers, such as poly(lactide acid) PLA, require sophisticated material processing to enable sufficient RF. and therefore are only applicable to small diameters. Other large-diameter stents show stent migration problems due to low RFs.
"W showed that rapid prototyped polymer stents could reach similar levels of RF compared with nitinol alternatives," they wrote. "FDM printing technology is an evolving and promising approach for polymer processing. It can be used for a broad range of thermoplastic materials where other rapid prototyping methods, such as laser sintering or stereolithography, offer fewer options due to their specific processing technique."
A downside of FDM's layered processing is the potential lower mechanical performance versus bulk material. Still, 3D printing was found to be an acceptable prototyping technique for an initial bioabsorbable stent design that offered a "good compromise" between material properties and feasibility to produce prototypes for further tests, the group wrote.
"Nowadays we are pursuing an alternative route to circumvent these limitations," Cabrera wrote. "We created porous structures that do not rely on struts to be expanded. This new type of stent can not only provide support and growth capacity, but also allows tissue producing cells to infiltrate and trigger a regenerative response that could heal the artery. With this promising approach, which relies on biocompatible and biodegradable materials, we could move forward with animal studies and we created a start-up company named STENTiT which has recently secured funding to continue improving the regenerative stent technology."
More information is available at the STENTiT website.