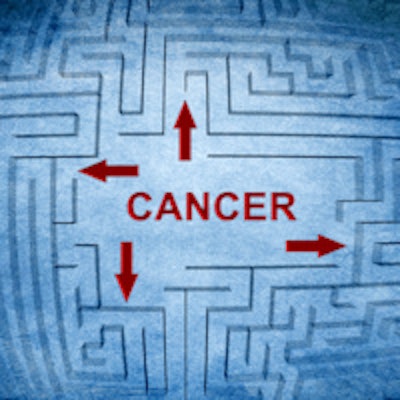
Proton therapy planning relies upon estimates of particle range in the patient, typically derived from a stoichiometric calibration that converts HU in an x-ray CT scan to relative stopping powers (RSP). This approach uses literature values of tissue composition, however, and patient-to-patient variations can result in RSP errors of more than 2%, placing a lower limit on treatment planning margins.
Addressing this, researchers at University College London (UCL) in the U.K., Massachusetts General Hospital (MGH) in Boston, and IBA in Louvain-La-Neuve in Belgium have devised a patient-specific calibration approach. Their strategy uses a proton radiograph to improve estimates of range that are quantified using water equivalent path lengths (WEPL).
"The ability to be able to more accurately predict WEPL will ultimately allow for tighter treatment planning margins and a greater sparing of normal tissues, reducing the toxicity and risk of secondary cancers to the patient," said Paul Doolan, first author and UCL physicist and PhD student.
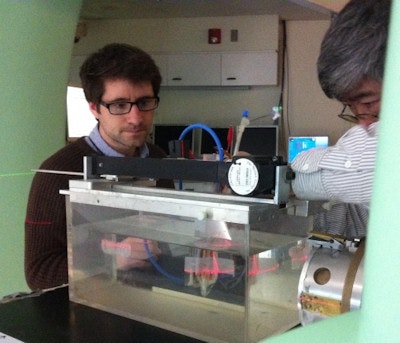
Proton CT can eliminate the need for calibration by providing 3D images of RSP directly, from which WEPL maps can be derived. However, the technique is not in routine use. Unlike x-rays, protons undergo multiple Coulomb scattering events on passing through tissue, limiting spatial resolution. Prototype CT scanners are also expensive, bulky, and could significantly impact clinical workflow, if part of a clinical treatment gantry. As a more feasible alternative, Doolan and his collaborators developed their hybrid approach (Physics in Medicine and Biology, February 2015, Vol. 60:5, 1901-1917).
"Combining [2D proton imaging] with a high-resolution x-ray CT dataset allows us to combine the benefits of both techniques: the geometric, anatomical information from the x-ray CT, and the RSP information that can be derived from a proton radiograph."
Iterative optimization
In the optimization, a WEPL map is derived from a proton radiograph taken in the planned direction of the therapy beam. The map is compared with an equivalent digitally reconstructed radiograph (DRR) created from the x-ray CT scan that also maps WEPL, rather than electron density. The DRR is generated by converting each voxel's HU to RSP using a generic stoichiometric calibration. Proton energy losses along ray traces through the imaged volume are then calculated where each ray trace corresponds to a pixel in the DRR.
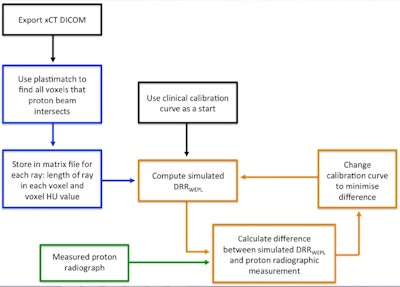
The generic calibration curve is then adjusted to reduce the difference between the two WEPL maps and reapplied to the x-ray CT data to construct a new DRR. The process is repeated until the difference between the two maps, quantified using a cost function, are minimized.
Improved WEPL accuracy
Using digital phantoms with known compositions and simulated images, the optimizer produced significantly more accurate WEPL values than those obtained with the generic calibration. The improvement was also seen in experimental measurements where the researchers acquired proton radiographs, along with x-ray CT scans, of two physical phantoms.
Developed by the MGH researchers with support from vendor Sun Nuclear, a simple system comprising a 2D array of 249 semiconductor diodes and the therapy beam as a proton source was used to acquire the radiographs. In a soft-tissue phantom, differences between WEPL maps generated from the calibrated x-ray CT data and directly from the proton radiograph were smaller after optimization. The root mean square error (RMSE) and mean difference were 2.9% and -0.2% with optimization, compared with 3.6% and -2.1% using the generic calibration.
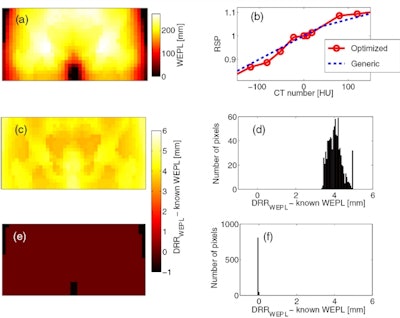
In a final test, the optimizer produced superior results when used to generate WEPL maps at a fixed, typical tumor depth in the same physical phantoms. Deviations from independently derived WEPL values calculated from depth-dose curves resulted in an RMSE of 1.4%, compared with 2.0% using the generic calibration in the soft-tissue phantom.
In future work, the collaboration plan is to adapt their technique for use with a scanned pencil beam and incorporate a higher-resolution proton detector. "Following successful development of the detector and the technique, we anticipate the first patients could be imaged in two to three years," Doolan said.
© IOP Publishing Limited. Republished with permission from medicalphysicsweb, a community website covering fundamental research and emerging technologies in medical imaging and radiation therapy.