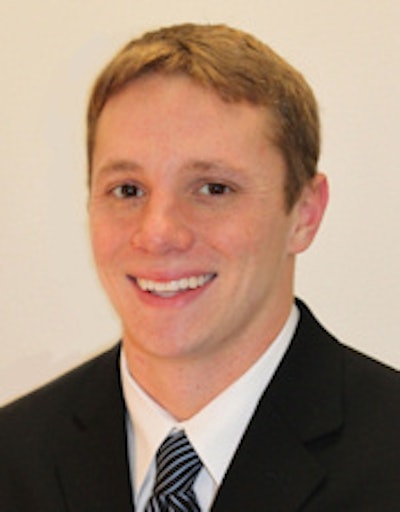
Measuring changes in tissue optical properties could provide a low-cost method for monitoring high-intensity focused ultrasound (HIFU), according to new research from the U.S. and U.K.
HIFU is a promising noninvasive alternative to open surgery for tumor resection and other ablative therapies. State-of-the-art HIFU procedures use MR thermometry to perform in vivo temperature measurements. A thermal dose model is then employed to relate the temperature profile to the probability of target tissue damage.
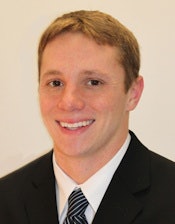
Recently, optical monitoring has been suggested as a lower-cost and simpler alternative for monitoring HIFU. As tissue is heated, damage to cellular membranes and proteins causes changes in its optical absorption and scattering properties, providing a direct indicator of any thermal damage.
"MR thermometry often works well for HIFU guidance, but there are many clinical scenarios where the expense of the process is unwarranted," explained Matthew Adams, a doctoral graduate student at Boston University in Massachusetts, U.S., who is currently undertaking a fellowship at the University of Oxford's Biomedical Ultrasonics, Biotherapy and Biopharmaceuticals Laboratory in the U.K. "Moreover, MR thermometry uses temperature measurements, which are an indirect way to infer thermal damage. Optical monitoring has the potential to provide an inexpensive, portable, real-time technology for directly sensing or imaging changes in optical properties during HIFU."
To investigate the relationship between thermal dose and tissue optical properties, Adams and colleagues studied thermally induced changes in the optical absorption coefficient, µa, and reduced scattering coefficient, µ's, of chicken breast samples. Their aim: to determine whether such changes can be accurately predicted using the standard thermal dose model (Physics in Medicine and Biology, 29 May 2014, Vol. 59:13, pp. 3249-3260).
Ex vivo investigations
The researchers examined 2-mm thick slices of chicken breast mounted between microscope slides. They used a UV-Vis-NIR spectrophotometer with an internal diffuse reflectance accessory to measure the total reflectance and transmittance of the samples between 500 and 1,100 nm, and employed the inverse adding-doubling algorithm to calculate µa and µ's from these measurements.
First, they studied the maximum thermally induced changes in the tissue's optical properties by immersing the samples in a heated saline bath at 50, 60, 70, 80, and 90° C until they reached a steady-state condition. Plotting µ's versus wavelength for the five temperatures confirmed that optical scattering increased with temperature, with a marked transition between 60° and 70° C.
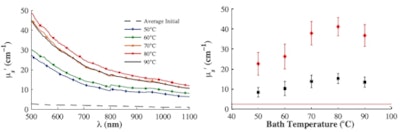
Plots of µ's at 550 and 975 nm (the deoxyhemoglobin and water absorption peaks) showed the transition between 60° and 70° C more clearly and suggests the existence of a threshold effect -- possibly due to a coagulation threshold for one of the proteins present in the chicken breast. The µa spectra also increased with exposure to high temperatures, but with no sign of a threshold within the investigated temperature range.
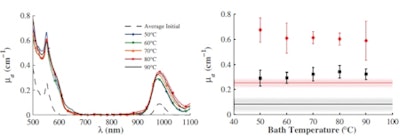
Adams and colleagues next investigated tissue changes as a function of thermal dose, by immersing the samples in the heated bath and measuring their temperatures with a thermocouple. They used the standard thermal dose model to calculate the thermal dose, which relates an arbitrary time-temperature profile, T(t), to the equivalent heating time at 43° C. For HIFU, the model calculates thermal dose by integrating R43-T(t) between initial and final temperatures, with the "isodose constant," R, taken as 0.5 above 43° C and 0.25 below.
The researchers measured the samples' optical properties at regular intervals, after removing them from the baths and bringing them to room temperature. Assuming that optical properties are linearly proportional to the fraction of damaged cells, they devised an equation to relate µa or µ's to thermal dose. The fitting parameters in this equation were t43, the time constant for heating at 43° C, and Dµ/µ0, the ratio of the maximum change in µa or µ's to their initial value.
Plots of scattering versus thermal dose revealed that Dµ's/µ's0 was relatively independent of bath temperature. However, t43varied by a factor of 20 over the different temperatures. For µa, t43 varied with bath temperature by up to 100-fold. These results imply that the standard thermal dose model used for most HIFU studies is insufficient for describing thermal changes in optical properties.
However, the researchers demonstrated that adjusting the isodose constant can lead to more consistent t43values. Using R = 0.63 above 43° C gave the smallest error between model and measured data. Refitting the scattering data using this adjusted isodose constant resulted in a t43 that varied by less than 40% over the studied temperatures and wavelengths. For µa, the values of t43were not constant, but the variation was significantly less than observed previously.
The authors concluded that the thermal dose model can capture changes in optical properties, but that the currently used isodose constant is not appropriate. They speculate that R is dependent upon (at least) the tissue type, rate of heating, and property of interest. Overall, scattering changes were significantly larger and more consistent than changes in absorption, suggesting that scattering may be the better property to use for HIFU monitoring.
"These results are currently being used to model optical changes during HIFU, in order to design and make predictions about the clinical viability of an acousto-optic-guided HIFU system," Adams said. "In the near future, we also hope to perform the same experiments in human tissue -- particularly in human breast tissue where acousto-optic-guided HIFU may be particularly viable."